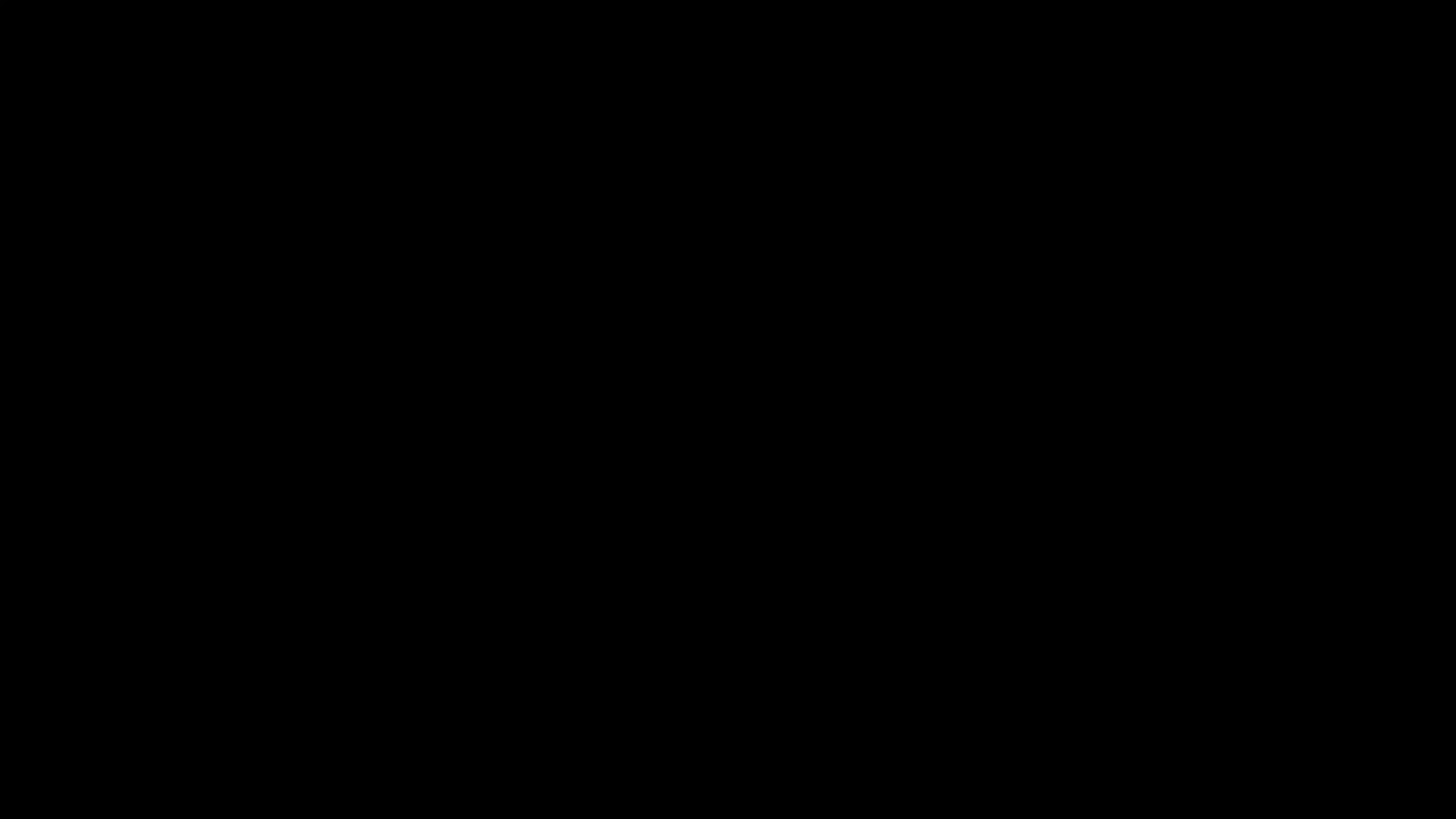
Bringing Scientific Insights into Focus
March 22, 2023
Science begins with observation. Whether it’s watching animals go about their daily activities or peering inside cells with a high-powered microscope, the simple act of looking can spark ideas, raise questions, and lead investigators down unexpected research paths. Sometimes the results are surprising. Following visual clues has led U of U Health investigators to new insights about how the brain learns, which parents’ genes shape behavior, and how cells protect themselves from potential toxins. In recognition of the power of seeing biology in action, an artistically inclined team of scientists is paving the way to future insights, with visual tools that bring unseen processes into focus.
Regardless of how they share their parenting duties, moms and dads make unique contributions that shape their offspring’s behavior. Some of that comes down to genetics. Because even though most genes are inherited in pairs, with each parent passing down one copy, the copies are not always used in the same way. Some genes are deployed differently depending on whether they came from mom or dad—and U of U Health researcher Christopher Gregg, PhD, has found in animal studies that this gives each parent distinctive roles in influencing mood and behavior.
One such gene encodes an enzyme that cells use to manufacture hormones and neurotransmitters that control these characteristics. Gregg and his colleagues discovered some brain cells use only the mother’s copy of this gene, while certain cells in the adrenal gland favor the father’s copy. By analyzing the movements of hundreds of mice as they foraged for food, the team uncovered a surprising consequence of this disparity in gene use: certain decisions in sons are controlled by their mother’s gene, whereas fathers have control over some decision-making in daughters. Understanding these complex genetics is a step toward developing better diagnoses and treatments for psychiatric conditions that involve the affected hormones and neurotransmitters, such as depression and addiction.
The neurons in our brains form billions of connections with one another—points at which information is passed from cell to cell. These connection points, or synapses, are not static. They change as we interact with the world. Synapses fade or become stronger in response to our experiences, changing the way information flows through our neural circuits. This is how we learn.
To speed up or slow down the exchange of information, cells can adjust the number of neurotransmitter receptors at a synapse. A single neuron may have thousands of synapses distributed along its long, branching projections. So how does it ensure that as we learn, receptors are delivered to the right places at the right times? U of U Health biologist Andres Villu Maricq, MD, PhD, and his colleagues have figured out how neurons manage this complex logistical problem. Maricq was able to visualize neurotransmitters moving through the neurons of worms during the learning process. His team’s experiments revealed cellular signals and structural scaffolds that control the loading of neurotransmitter receptors onto the molecular motors that ferry them to their destinations. Their findings improve scientists’ understanding of learning and memory at the cellular level.
Amino acids are one of the most fundamental building blocks of life, and our bodies require a steady supply to keep everything working as it should. But when supply exceeds demand, amino acids that go unused can be harmful to cells. Levels can fluctuate as we take in protein through our diet, and it’s up to cells to adapt and adjust. While it’s not yet clear how excess amino acids cause damage, elevated levels in the blood have been linked to several diseases, including cancer and diabetes.
After noticing unexplained structures emerging inside aging yeast cells, U of U Health biochemist Adam Hughes, PhD, and his colleagues discovered a surprising way that cells protect themselves from excess amino acids. They discovered that cells construct those structures, which they called mitochondrial-derived compartments, when amino acid levels get too high. Their presence promotes amino acid breakdown and impedes the synthesis of new amino acids by tucking away key nutrient transporters. By elucidating how cells keep their amino acids within healthy levels, the Hughes’ lab is setting the stage for understanding how and why that balance is sometimes lost.
When the SARS CoV-2 virus emerged in 2019, scientists and health care workers scrambled to fight an invisible foe. Within months, researchers captured the first snapshots of the spike-studded microbe, and it quickly became recognizable around the world. Further research brought the threat into even sharper focus, revealing insights that helped scientists and governments better anticipate the virus’s behavior, improve public health strategies, and investigate potential treatments.
Knowing how vital it is to see the virus in action, U of U Health investigator Janet Iwasa, PhD, and colleagues have created a vibrant animation that pulls together a wealth of information about the life cycle of SARS CoV-2. Viewers can follow the virus with molecular-level detail as it enters and hijacks human cells. The animations represent a working model of SARS CoV-2 infection based on current knowledge, helping to clarify which aspects of coronavirus biology are well understood and where more research is needed. Their interactive online interface incorporates annotated descriptions of the data behind the visualizations and encourages discussion within the research community.
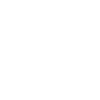
Pioneering the Future: Stories of Discovery & Innovation at University of Utah Health
Special thanks to Wes Sundquist and Alfred Cheung for their work to compile the discoveries and innovations that make this series possible.
Written by: Jennifer Michalowski
Editing by: Julie Kiefer
Layout and Design by: Kyle Wheeler
Production Supervision: Abby Rooney, Julie Kiefer, Kyle Wheeler
Supported by: Rachel Hess, Chris Hill, Amy Tanner